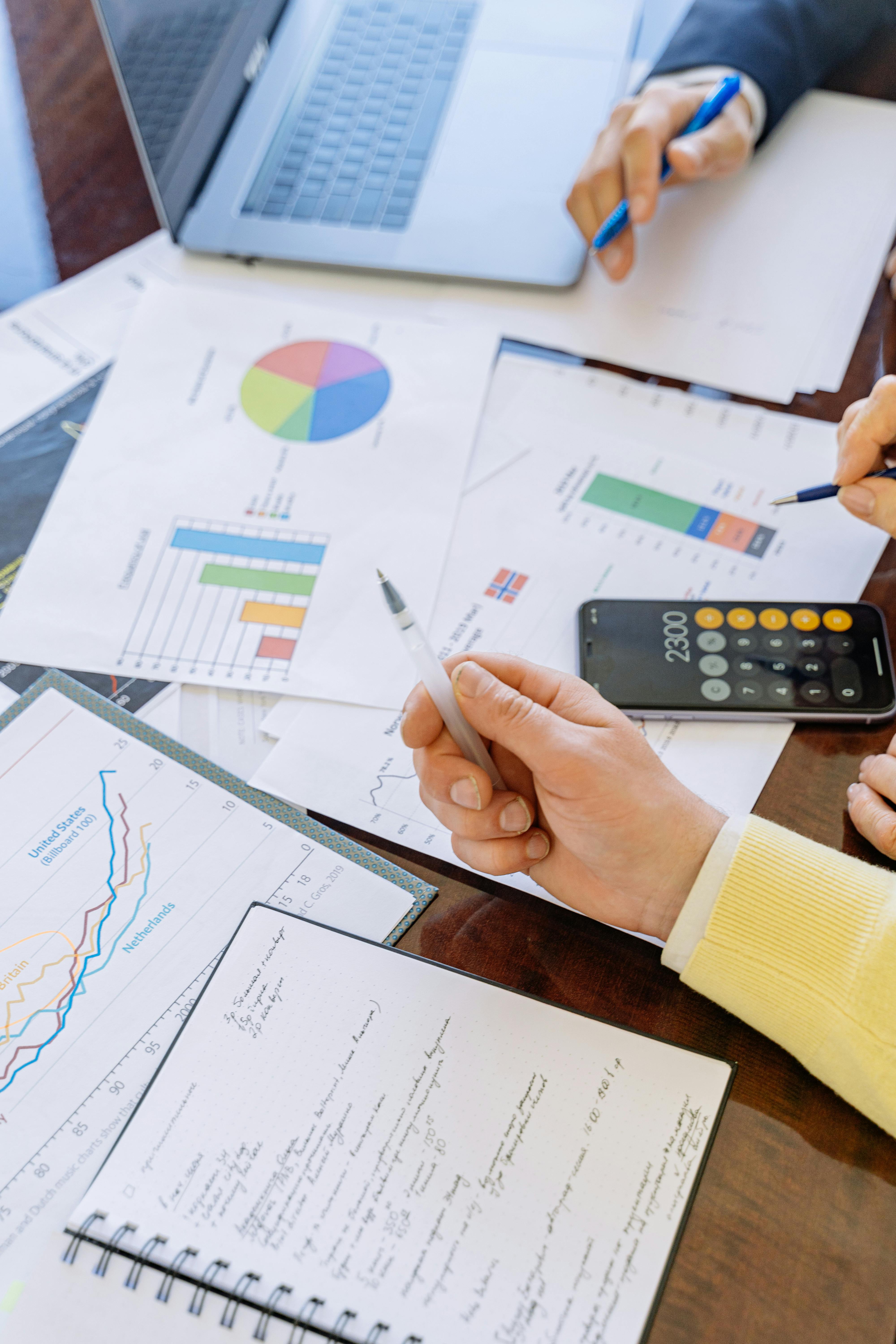
Essential Guide to How to Find Limiting Reactant in Chemistry
Understanding how to find the limiting reactant is central to mastering stoichiometry in chemistry. This essential guide dives deep into the topic, exploring practical applications and advanced techniques in determining limiting reagents in chemical reactions. Whether you're preparing for lab experiments or solving complex stoichiometric calculations, this article provides all the necessary tools and insights.
What is a Limiting Reactant?
The concept of a limiting reactant plays a crucial role in chemistry. In any chemical reaction, a limiting reactant is the substance that runs out first, thus determining the maximum amount of products formed. It is essential to identify the limiting reactant because it helps in calculating the theoretical yield and understanding the reactants' efficiency. To illustrate, consider combining hydrogen gas and oxygen gas. The balanced equation indicates the necessary stoichiometric ratio: 2H₂ + O₂ → 2H₂O. If you start with 4 moles of H₂ and only 1 mole of O₂, the O₂ limits the reaction to 2 moles of water, rendering it the limiting reactant. Proper identification allows for accurate predictions of reaction outcomes.
Identification of the Limiting Reactant
To identify the limiting reactant in a chemical equation, you can follow a systematic approach. Begin with a balanced equation. For the previous example, ensure the reaction representing hydrogen and oxygen is accurately balanced. Then, calculate the number of moles for each reactant present. Next, use the molar ratio outlined in your balanced equation to determine how much reactant is needed. For instance, you need 2 moles of H₂ for every mole of O₂. If calculations reveal insufficient moles of one reactant, that is your limiting reactant. This method ensures precision in experimental design and sets the stage for successful quantitative analysis.
Calculations and Molar Ratios
A molar ratio is fundamental in the determination of the limiting reactant. To calculate yields and reactant limits, start by quantifying the moles of each reactant in a reaction based on the molecular weight of each substance. Suppose you have 10 grams of H₂ and 32 grams of O₂. The molar mass of H₂ is approximately 2 g/mol, giving you 5 moles, and O₂ has a molar mass of approximately 32 g/mol, yielding 1 mole of O₂. Using stoichiometric coefficients from the balanced equation, determine which reactant produces the least product. The calculations will reveal which reactant is in surplus and support precise decisions in further laboratory techniques.
Practical Applications of Limiting Reactant Calculations
The concept of identifying the limiting reactant extends beyond theoretical concepts into practical applications in laboratory settings and chemical synthesis. For chemists and students alike, mastering the process enhances accuracy and yields. In many laboratory settings, chemical processes must be monitored closely to ensure that resources are effectively utilized and waste is minimized. Correctly identifying the limiting reactant allows chemists to achieve maximum efficiency and aids in reaction completion.
Case Studies in Laboratory Techniques
Laboratory experiments often utilize limiting reactant principles to gauge reactant efficiency. For example, in a lab experiment involving the synthesis of water, consider using 4 grams of hydrogen and 32 grams of oxygen. After initial setup and calculations, determine the limiting reactant and optimize conditions, such as temperature and pressure, to enhance the reaction rate. Post-experimentation, yield your findings and analyze the results, confirming the theoretical yield is reflected in actual measurements. Using quantitative analysis, such as a spectrophotometer, can help visualize the impact of changing variables, granting valuable insights into the dynamics of chemical reactions.
Improving Yield with Reaction Pathway Considerations
Understanding the reaction pathway also influences how one approaches limiting reactants. Beyond mere calculations, chemists explore reaction kinetics to grasp how changes in concentrations can shift the way products are formed. For instance, employing an enzyme in a blend of reactants may alter limiting factors. By understanding the full implications of the limiting reactants in terms of reaction kinetics, chemists can manipulate conditions for improved outcomes, ultimately enhancing the percent yield of desired products.
Common Errors in Limiting Reactant Calculations
When conducting stoichiometric calculations, ensuring accuracy in measuring reactants is vital. Misestimating reactant quantities often leads to errors in determining the limiting reactant. Therefore, constructive practices, such as repeated vigilance while measuring and adhering to proper laboratory safety protocols, will support robust results. Moreover, familiarity with stoichiometric calculations and common pitfalls can safeguard against yielding improper conclusions about reactants' roles.
Measurement Precision and Computational Approaches
Precision is paramount. When working in a lab, employing electronic scales and precise volumetric flasks promotes measurement accuracy, essential for reliable outcomes in stoichiometric calculations. Further, incorporating computational tools can aid scientists by instantly performing iterative analyses through simulation software that accurately tracks reaction intermediates and calculates expected yields. Such advanced approaches not only enhance physically conducted experiments but also mitigate errors associated with computational data.
Troubleshooting Techniques in Laboratory Settings
If discrepancies arise between theoretical and actual yields, systematic troubleshooting helps clarify miscalculations. For instance, if expected outcomes are not realized, re-evaluate reactant handling techniques and assess potential side reactions that could diminish product yield. By examining all chemical behaviors and ensuring reagent preparation stays consistent, chemists enhance overall reactions and problem-solving techniques within their practices. Furthermore, keeping accurate laboratory documentation allows for better identification of errors and aids in refining methods for future experiments.
Key Takeaways
- Identification of the limiting reactant is essential to accurately predict product yields.
- Utilize molar ratios and balanced equations to guide calculations and enhance understanding.
- Practical applications involve optimizing laboratory techniques for better yield efficiency.
- Measurement precision and troubleshooting methodologies significantly affect experimental success.
FAQ
1. What is the importance of determining the limiting reactant?
Determining the limiting reactant is crucial because it directly affects the amount of product generated in a chemical reaction. Understanding this concept ensures that chemical synthesis and laboratory experiments are optimized for yield and efficiency.
2. How do you know if you've balanced a chemical equation correctly?
A balanced chemical equation will have equal numbers of each type of atom on both sides. You can verify this by ensuring that the coefficients match across the reaction. For example, if you have 2H₂ + O₂ → 2H₂O, check that the number of H and O atoms is equal on both sides.
3. What are the typical errors in stoichiometric calculations?
Common errors include miscalculation of mole quantities, inaccurate measurements, and misinterpretation of the balanced equation. Ensuring precision in laboratory practices can minimize these risks effectively.
4. Can real-life applications of limiting reactant concepts be illustrated?
Yes! Industries involved in chemical manufacturing frequently apply limiting reactant principles to maximize product output and minimize waste. This direct application underscores the importance of theoretical concepts in practical chemistry.
5. How does reaction rate affect the limiting reactant?
The reaction rate can influence which reactant is limiting, as faster reactions may consume certain reactants more quickly than expected. Monitoring reaction conditions closely can help predict outcomes and adjustments necessary for optimal efficacies.